NORTHUMBRIA UNIVERSITY, NEWCASTLE UPON TYNE, UK
PAST FUEL RESEARCH
PREmixed Mixture Ignition in the End-gas Region (PREMIER) new combustion mode
This mode of combustion has been investigated in previous studies with natural gas, and has been given the name PREmixed Mixture Ignition in the End-gas Region (PREMIER) combustion. PREMIER combustion begins as premixed flame propagation, and then, because of mixture autoignition in the end-gas region, ahead of the propagating flame front, a transition occurs, with a rapid increase in the heat release rate. It was determined that the mass of fuel burned during the second stage affected the rate of maximum pressure rise. As the fuel mass fraction burned during the second stage increased, the rate of maximum pressure rise also increased, with a gradual decrease in the delay between the first increase in the heat release rate following pilot fuel injection and the point when the transition to the second stage occurred. The H2 and CO2 content of syngas affected the engine performance and emissions. Increased H2 content led to higher combustion temperatures and efficiency, lower CO and HC emissions, but higher NOx emissions. Increased CO2 content influenced performance and emissions only when it reached a certain level. In the most recent studies, the mean combustion temperature, indicated thermal efficiency, and NOx emissions decreased only when the CO2 content of the syngas increased to 34%. PREMIER combustion did not have a major effect on engine cycle-to-cycle variation. The coefficient of variation of the indicated mean effective pressure (COVIMEP) was less than 4% for all types of fuel at various equivalence ratios, indicating that the combustion was within the stability range for engine operation.
PREMIER combustion concept was developed at Heat Power Engineering Lab of Okayama University, Japan. The team was supervised by Prof. Eiji Tomita and Prof. Nobuyuki Kawahara.
Below is conceptial illustration of PREMIER combustion concept.

The transition from PREMIER combustion to knocking combustion was evaluated by FFT analysis of the in-cylinder pressure and the knock-sensor signals. Unlike knocking in traditional SI engines, high-frequency oscillations of in-cylinder pressure and knock-sensor signals were not observed during the PREMIER combustion mode.

As shown in figure below, OH* peaks of PREMIER combustion at 12 deg. and 15 deg. ATDC have the same magnitude.The reason for the same magnitude in OH* is that the maximum rate of pressure rise is slower than that during the knocking case.
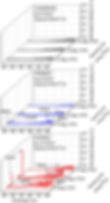
As shown in figure below, PREMIER combustion can be maintained within a wide range of pilot fuel injection pressures.

Figure below indicates that PREMIER combustion may pass through different IMEP levels, depending on the equivalence ratio and injection timing. As the H2 fraction of the syngas increased (from Type 1 to Type 2), the PREMIER combustion region expanded at lower equivalence ratios and shrunk at higher equivalence ratios.
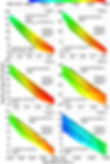
Modeling of flame lift-off length in diesel low-temperature combustion
Low-Temperature Combustion (LTC) is becoming a promising technology for simultaneously reducing soot and NOx emissions from diesel engines. LTC regimes are evaluated by the flame lift-off length - the distance from the injector orifice to the location of hydroxyl luminescence closest to the injector in the flame jet. Various works have been dedicated to successful simulations of lifted flames of a diesel jet by use of various combustion modeling approaches. In this work, flame surface density and flamelet concepts were used to model the diesel lift-off length under LTC conditions. Numerical studies have been performed with the ECFM3Z model, n-Heptane and diesel fuels to determine the flame lift-off length and its correlation with soot formation under quiescent conditions. The numerical results showed good agreement with experimental data, which were obtained from an optically accessible constant volume chamber and presented at the Engine Combustion Network (ECN) of Sandia National Laboratories. It was shown that at a certain distance downstream from the injector orifice, stoichiometric scalar dissipation rate matched the extinction scalar dissipation rate. This computed extinction scalar dissipation rate correlated well with the flame lift-off length. For the range of conditions investigated, adequate quantitative agreement was obtained with the experimental measurements of lift-off length under various ambient gas O2 concentrations, ambient gas temperatures, ambient gas densities and fuel injection pressures. The results showed that the computed lift-off length values for most of the conditions lay in a reasonable range within the quasi-steady lift-off length values obtained from experiments. However, at ambient temperatures lower than 1000 K, the lift-off length values were under-predicted by the numerical analysis. This may be due to the use of the droplet evaporation model as it is believed that evaporation has a strong effect on the lift-off length.
To determine the scalar dissipation rate along the stoichiometric line of mixture fraction, the iso-surface of mixture fraction values at a specific stoichiometry was created, as shown in figure (A) below. The red contour in figure (A) is a stoichiometric mixture fraction line at different O2 concentrations.
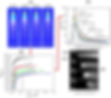
Visualization of flame luminocity of GTL and GTL-Biodiesel fuel blends
An experimental study has been performed on spray combustion and two-dimensional soot concentration in diesel (ULSD), GTL and GTL-biodiesel fuel jets under high-pressure, high-temperature quiescent conditions. Instantaneous images of the fuel jets were obtained with a high-speed camera. It was confirmed that by blending GTL with 20% rapeseed biodiesel, certain fuel properties such as kinematic viscosity, density, surface tension, volatility, lower heating value and others may be designed and improved to be more like those of conventional diesel fuel but with considerable decrease in the amount of sulfur, PAH, cold filter plugging point, etc. The results showed that the spray tip penetration increased and the spray cone angle decreased when 20% biodiesel fuel was added to GTL fuel. Autoignition of the GTL-biodiesel blend occurred slightly earlier than that of diesel fuel. Experiments under high-pressure, high-temperature conditions showed that higher injection pressure induced a lower soot formation rate. The integrated flame luminosity, which serves as an indicator of soot concentration in the fuel jet, was slightly higher for the GTL-biodiesel blend than for pure GTL fuel due to the slightly higher sulfur content of pure biodiesel fuel.
Fuels used in this study: (A) ULSD; (B) GTL; (C) biodiesel;
(D) G80-B20.

The integrated flame luminosity of G80-B20 was slightly higher than that of GTL due to the slightly higher sulfur content in the pure biodiesel fuel. The fuel-bond sulfur is a source of PAHs, which are soot precursors.
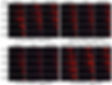
Visualization of soot formation in diesel-GTL jet with shadograph method
In this study, a visual investigation of sprays and flames is performed, and soot formation in Diesel-GTL fuel blends is studied in a specially designed quiescent constant-volume chamber under various ambient gas temperatures and O2 concentrations. Similar to the case of soot formation during diesel fuel combustion, the sooting zone during the mixingcontrolled combustion of Diesel-GTL blends is located in the leading portion of the jet boundaries. Auto-ignition delay and soot concentration decrease with an increase of GTL content in the fuel blend. Soot also decreases with lower O2 concentration, higher injection pressure, and lower ambient gas temperature. The lack of soot formation at lower O2 concentrations and lower temperatures suggests that Diesel-GTL fuel blends can be successfully utilized in low-temperature diesel combustion technologies that are currently being developed. Furthermore, this mixing controlled combustion method with Diesel-GTL blends can be used to modulate various engine operation parameters, and therefore to simultaneously reduce the formation of soot and NOx within a wide range of diesel engine loads.
The shadowgraph images of regions of soot formation in the fuel blend jets show distinct density gradients formed in the leading portion of the jet boundaries. Soot formation appears first in the region near the spray tip due to the fuel-rich premixed burn. As the combustion becomes purely mixing-controlled, no soot cloud is observed. In fact, it has been a concern that the soot emission level may increase due to the decrease in the ignition delay period. As is seen, however, even though the.
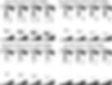
Dual-fuel combustion with syngas
To satisfy increasingly strict emissions regulations, synthesis gas appear to be greener alternatives for internal combustion engines. In many situations where the price of petroleum fuels is high or where supplies are unreliable, the syngas, for example, can provide an economically viable solution. Syngas is produced by gasifying a solid fuel feedstock such as coal or biomass. The biomass gasification means incomplete combustion of biomass resulting in production of combustible gases. Syngas consists of about 40% combustible gases, mainly carbon monoxide (CO), hydrogen (H2) and methane (CH4). The rest are non-combustible gases and consists mainly of nitrogen (N2) and carbon dioxide (CO2). Varying proportions of CO2, H2O, N2, and CH4 may be present.
H2 as a main component of a syngas has very clean burning characteristics, a high flame propagation speed and wide flammability limits. H2 has a laminar combustion speed about eight times greater than that of natural gas, providing a reduction of combustion duration and as a result, an increase in the efficiency of internal combustion (IC) engines, if the H2 content in the gaseous fuel increases. Main point of interest in increasing H2 content in the gaseous fuel is that with the addition of H2, the lean limit of the gas operation can be extended, without going into the lean misfire region. Lean mixture combustion has a great potential to achieve higher thermal efficiency and lower emissions. In particular, the lean mixture combustion will result in low and even extremely low NOx levels with only a slight increase in hydrocarbons.
Figure below shows the effect of pilot diesel fuel amount on combustion of syngas as a primary fuel. A distinct separation of diesel diffusion flame and syngas premixed flame is seen when larger amount of diesel fuel, 10 mg, is injected (A). The mixture burns only when the required oxygen is present. As a result, the flame speed is limited by the rate of diffusion. For syngas well-premixed mixture, the flame is not limited by the rate of diffusion and the mixture burning rate is much faster than that of diesel-air mixture. When only 2 mg of diesel fuel is injected (B) the fuel evaporates and mixes with the oxidizer much faster providing distributed ignition centers in the cylinder for syngas-air mixture which is burnt as a premixed flame propagating towards the cylinder wall.

The image processing enabled to detect early ignition kernels when diesel-air mixture is autoignited and consequently ignites syngas.

Dual-fuel combustion modeling with syngas
A multidimensional computational fluid dynamics (CFD) simulation of a constructed syngas chemical kinetic mechanism was performed to evaluate the combustion of syngas in a supercharged dual-fuel engine for various syngas initial compositions under lean conditions. The modelled results were validated by comparing predictions against corresponding experimental data for a supercharged dual-fuel engine. The predicted and measured in-cylinder pressure, temperature, and rate of heat release (ROHR) data were in good agreement. The effect of the hydrogen peroxide chain-propagation reaction on the progress of combustion under supercharged conditions was examined for different types of syngas using various initial H2 concentrations. The effect of the main syngas kinetic mechanism reactions on the combustion progress was analysed in terms of their contribution to the total heat of the reaction. The best results compared with experimental data were obtained in the range of equivalence ratios below about 0.8 for all types of syngas considered in this paper. As the equivalence ratio increased above 0.8, the results deviated from the experiment data. The spatial distribution of the in-cylinder temperature and OH* within this equivalence-ratio range showed the completeness of the combustion. The present CFD model captured the overall combustion process well and could be further developed into a useful tool for syngas-engine combustion simulations.
The model predicted the engine performance well, including the cylinder pressure history, heat-release rate data with respect to syngas composition equivalence ratio, and injection timing. The results demonstrated that under supercharged engine conditions, the importance of H2O2-based reactions should not be underestimated.

Formaldehyde formation and its effect on combustion
Experiments were conducted in a compression–expansion test engine to investigate the combustion characteristics in a homogeneous charge compression ignition (HCCI) engine fuelled with dimethyl ether.
Two types of analyses were performed. In the first, ultraviolet–visible (UV–Vis) light absorbance was investigated to identify the formation behaviour of HCHO and OH during HCCI combustion. In the second, knocking combustion was investigated by analysing the spatially integrated flame luminosity and in-cylinder pressure oscillations. The time-resolved HCHO and OH profiles at different equivalence ratios showed that HCHO absorbance increased in the low-temperature reaction (LTR) and thermal-preparation regions and gradually decreased as the combustion approached the high-temperature reaction (HTR) region. The in-cylinder temperature in the LTR region had little effect on the rate of the maximum pressure rise, and this did not change much at different equivalence ratios. The results demonstrated that there was a marked difference between the intensity of the flame emissions of non-knocking and knocking events. The time-resolved integrated absorbance spectra of HCHO with peaks at 328, 340, and 354 nm that occurred before the OH peaks appeared suggested that when a certain threshold ratio of (dP/dhLTR)/(dP/dhHTR) was reached, the amount of HCHO decreased due to reactions in the thermal-preparation region while the tendency to knock increased.
Figure below shows time resolved formaldehyde spectra over a broad range of wave lengths.
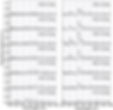
Figure below shows the time-resolved HCHO and OH profiles during the DME–O2–Ar mixture combustion at different equivalence ratios. The HCHO absorbance increased during the LTR and thermal- preparation regions and gradually decreased as the combustion approached the HTR region.The opposite trend was observed for the OH absorbance profiles: the OH was at a minimum in the LTR region and increased as the combustion approached the HTR region.
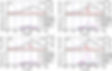
The possible effect of HCHO on knocking, as shown in figure below, suggested that HCHO increased at a lower RI until the moment when the (dP/dhLTR)/(dP/dhHTR) ratio reached a certain threshold. As this ratio decreased, the amount of HCHO was reduced due to reactions in the thermal-preparation region, with a corresponding release of a considerable amount of heat as HCHO was consumed.
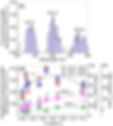
Large-Eddy Simulation (LES) of diesel spray combustion
Large-Eddy Simulation (LES) was used to perform computations of air entrainment and mixing during diesel spray combustion. The results of this simulation were compared with those of Reynolds Averaged Navier Stokes (RANS) simulations and an experiment. The effect of LES on non-vaporizing and vaporizing sprays was evaluated. The validity of the grid size used for the LES analysis was confirmed by determining the subgrid-scale (SGS) filter threshold on the turbulent energy spectrum plot, which separates a resolved range from a modeled one. The results showed that more air was entrained into the jet with decreasing ambient gas temperatures. The mass of the evaporated fuel increased with increasing ambient gas temperatures, as did the mixture fraction variance, showing a greater spread in the profile at an ambient gas temperature of 920K than at 820 K. Flame lift-off length sensitivity was analyzed based on the location of the flame temperature iso-line. The results showed that for the flame temperature iso-line of 2000oC, the computed lift-off length values in RANS matched the experimental values well, whereas in LES, the computed lift-off length was slightly underpredicted. The apparent heat release rate (AHRR) computed by the LES approach showed good agreement with the experiment, and it provided an accurate prediction of the ignition delay; however, the ignition delay computed by the RANS was underpredicted. Finally, the relationships between the entrained air quantity and mixture fraction distribution as well as soot formation in the jet were observed. As more air was entrained into the jet, the amount of air-fuel premixing that occurred prior to the initial combustion zone increased, upstream of the lift-off length, and therefore, the soot formation downstream of the flame decreased.
Figure below shows comparisons of the vaporizing spray penetrations for the SGS k-Δ and RANS models with that of the experiment. At the earlier time steps, SGS k-Δ overpredicted the spray penetration, but as time elapsed, the computed spray penetration matched closely with that of the experiment; on the other hand, the RANS simulation underpredicted the spray penetration at the later time steps.

Figure below shows that although the mixture fraction scalar distribution is wider for higher injection pressures, the magnitude of the mixture fraction variance is lower. This finding may be explained by the fact that the higher fuel injection pressure causes more intense fuel atomization and breakup and wider liquid-vapor fuel distribution within the chamber.

Figure below shows the instantaneous images of the experimental spray flame distributions and the temperatures, mixture fractions, soot and NOx scalars computed by LES.
